Keynotes 2012
Keynote papers for 2012
Thin Film Evaporative Cooling in Microgap Channels

Prof. Avram Bar-Cohen
University of Maryland
Abstract: Two-phase flow of coolants, undergoing phase change while flowing in microgap channels and tubes, is a most promising - though as yet poorly understood - technique for a wide range of thermal management and energy conversion applications. Microgap coolers are of particular interest in the thermal packaging of high flux semiconductor chips, chip stacks, and other microelectronic components in which high heat fluxes are accompanies by high heat densities. In such microgap channels Annular flow - associated with the presence of a thin liquid layer flowing along the outer wall of the channel and a vapor core in the center - has been found to be the dominant flow regime. Moreover, since the microgap dimension dictates vanishingly thin, "annular" liquid films, the low thermal resistances to diffusion and the ease of evaporation into the vapor core can result in substantial evaporative heat transfer rates at the wetted walls. This presentation will open with a brief discussion of two-phase flow regimes in microgap channels and the M-Shaped heat transfer coefficient variation induced by the axial regime progression. The reported microgap heat transfer coefficients, sorted by the prevailing flow regimes, will be compared to predictions from classical two-phase correlations. For the high-quality, Annular flow conditions, the venerable Chen correlation is shown to yield predictive agreement for the heat transfer coefficients in microgap channels that is comparable to that attained for macrochannels and to provide a mechanistic context for the thermal transport rates attained in microgap channels. Attention will then be turned to the deterioration in the local evaporative heat transfer coefficient that follows the second peak in the M-shaped axial variation. Results obtained from infrared imaging, revealing previously undetected, large surface temperature variations in Annular flow, are also reviewed and related to the termination of the favorable thin-film evaporation mode in such channels. The parametric dependence of these fluctuations on the microgap channel width, height, and length, as well as working fluid, is also discussed, along with the observed effect of channel orientation, on the average and local heat transfer coefficients in microgap channels.
This work conducts a review on the heating of nanoparticles by an external source such as ultrasound, laser, microwave, magnetic field and electromagnetic field at RF spectrum, and reports our latest development in the field, especially the ultrasound and RF heated nanoparticle suspensions for targeted medical applications.
Dr. Avram Bar-Cohen is an internationally recognized leader in the development and application of thermal science and engineering to microelectronic and optoelectronic systems. In his role at the Defense Advanced Projects Agency (DARPA) and through his professional service in IEEE and ASME, he has defined and guided the field of thermal packaging and facilitated the emergence of high reliability consumer electronics, computing platforms, and microwave communication and radar systems. His research and publications, as well as short courses, tutorials, and keynote lectures, on air-cooled card cages and heat sinks, along with direct liquid cooling of microprocessors and RF components, have helped to create the scientific foundation for the thermal management of micro- and nanoelectronic components and systems.
He is an Honorary member of ASME, and Fellow of IEEE, as well as Distinguished University Professor in the Department of Mechanical Engineering at the University of Maryland. From 2001 to 2010 he served as the Chair of Mechanical Engineering at Maryland. Bar-Cohen’s honors include the Luikov Medal from the International Center for Heat and Mass Transfer in Turkey (2008), ASME’s Heat Transfer Memorial Award (1999), Curriculum Innovation Award (1999), Edwin F. Church Medal (1994) and Worcester Reed Warner Medal (2000), and the Electronic and Electrical Packaging Division’s Outstanding Contribution Award (1994) as well as the InterPack Achievement Award (2007). Bar-Cohen was the founding chair of the IEEE Intersociety Conference on Thermal Management in Electronic Equipment (ITHERM) in 1988 and was recognized with the IEEE CPMT Society’s Outstanding Sustained Technical Contributions Award (2002), the ASME/IEEE ITHERM Achievement Award (1998) and the THERMI Award from the IEEE/Semi-Therm Conference (1997).
Bar-Cohen has co-authored Design and Analysis of Heat Sinks (Wiley, 1995) and Thermal Analysis and Control of Electronic Equipment (McGraw-Hill, 1983), and has co-edited 16 books in this field. He has authored/co-authored some 400 journal papers, refereed proceedings papers, and chapters in books; has delivered 65 keynote, plenary and invited lectures at major technical conferences and institutions, and he holds 8 US and 3 Japanese patents. He has advised to completion 64 PhD and master’s students at the University of Maryland, the University of Minnesota and the Ben Gurion University (Beer Sheva, Israel), where he began his academic career in 1972. From 1998-2001 he directed the University of Minnesota Center for the Development of Technological Leadership and held the Sweatt Chair in Technological Leadership.
Teaching Heat Transfer and Fluid Flow by Means of Computational Fluid Dynamics (CFD)

Prof. Brian Spalding
Concentration Heat & Momentum (CHAM) Limited, Wimbledon Village, London, England
Abstract: What? This lecture considers alternative teaching methods in the fields of heat transfer and fluid flow. Traditional teaching of heat transfer and fluid flow relies heavily on the student's abilities to (a) perform certain mathematical operations, and (b) comprehend their consequences in physical terms. The mathematical operations are of the 'functional-analysis' kind, involving differential equations, the solutions of which can be expressed in terms of commonly-used functions: trigonometric, hyperbolic, error, Bessel etc. The lecturer argues that modern-times teachers should reduce the emphasis on functional analysis and exploit the less-limited power of numerical analysis. Why? Traditional teaching methods have limited success because: (1) they cannot teach students who, although possessing other abilities more conducive to success as practising engineers, are weak in respect of one or other of abilities (a) and (b) above: (2) they can handle only idealised physical situations, of simplified geometry, material properties and flow-defining conditions, which are seldom encountered in engineering practice. After graduation, engineers must therefore perforce make use of numerical analysis to perform their professional tasks of equipment and process design; and they do so with more success if, as students, they have learned the merits and the drawbacks of computational fluid dynamics. Practising engineers in fact make scant use of the abilities (a) and (b), weakness in which has excluded from their profession many who could have succeeded perfectly well in it. The lecturer argues that his proposal therefore has not-inconsiderable social implications; for CFD, when used as a teaching tool, has graphical-display capabilities which render its consequences very easy to comprehend in physical terms. How Traditional-style teachers use traditional-style text-books because they are available; whereas the equivalent CFD-based tools are not (yet). Available general-purpose CFD codes could in principle be used; but only after immense labour; for the necessary 'middle-ware' has been lacking. The present lecture argues that, when what is needed is carefully distinguished from what is not, the middle-ware is not hard to create; and by the teachers themselves rather than less-likely-to-be-interested CFD specialists. Examples of 'simlets' (ie small simulation packages) will be described and demonstrated. These focus on text-book-chapter-sized topics (eg pipe flow, finned surfaces, radiative heat exchange). They show conformity with functional analysis for the idealised situations to which that can be applied; but they show how numerical analysis can handle practical situations which functional analysis can not. Simlets do make use of general-purpose computer codes as their subterranean engines; but neither teacher nor student needs to be aware of the fact. No computer more powerful than a 'netbook' is required; and 'Kindle-style' hardware will no doubt be offered when the simlet notion becomes widely appreciated. When The lecturer argues that the time to exploit the new capabilities is now; and, not himself having very much time left, will welcome collaborators who share his desire to produce as many 'simlets for teachers' as possible in the next very few years.
Brian Spalding is a founding father of computational fluid dynamics (CFD) having started to work on it at Imperial College, London, in the late 1960’s and consolidating his activities in the early 1970’s. He received the Franklin Medal in 2010 for “his seminal contributions to the computer modelling of fluid flow, creating the practice of computational fluid dynamics (CFD) in industry, and paving the path for the widespread application of CFD to the design of objects from airplanes to heart valves.”
Whilst Professor of Heat Transfer and Head of the CFD Unit at Imperial College he founded Concentration Heat and Momentum (CHAM) Limited which was the first commercial company to provide CFD consulting services nationally and internationally. It was with his colleagues at CHAM that Spalding developed PHOENICS which, in 1981, was the first commercially disseminated CFD code and which is the basis of many, if not all, of the commercially available software used today.
Spalding came to CFD via a general interest in engineering science at Oxford; combustion (his PhD work at Cambridge was on the combustion of liquid fuels); the modelling of heat transfer two-phase and multiphase flow, chemical reaction and stresses in solids; turbulence (his turbulence models from the 1970’s are still in use) and an early understanding of the potential of computers. He is still interested in scientific matters outside CFD, for example he is currently part of a team re-writing the Heat Exchanger Data Handbook.
He is active in his London-based company and, in addition, spends time in Moscow where he works with a small research group. He has inspired students and others in the course of his scientific life and they, in their turn, have inspired their students. Spalding’s students are retiring now and it is the ‘grandchildren’ who are working on ideas to solve problems using his SIMPLE algorithm, IPSA or PHOENICS. New versions of these algorithms, and of his computer code, will come from younger generations but Spalding himself is still interested in new ideas as can be seen by the subject matter of the current lecture.
Multi-phase Fluid Behaviour and Thermal Characteristics in Flow Through Heated Curved Ducts

Prof. Tilak Chandratilleke
Department of Mechanical Engineering, Curtin University, Western Australia
Abstract: Owing to the centrifugal action imparted by duct curvature, curved ducts have unique spiralling axial flow behaviour that is fundamentally different to those in straight passages. Within curved passages, the centrifugal forces induce on the axial flow a lateral fluid movement, known as secondary flow, which is characterised by pairs of counter-rotating vortices. The secondary flow is susceptible to hydrodynamic instability beyond certain fluid flow rates, that is manifested by the emergence of additional pairs of secondary (or Dean) vortices in the flow. The secondary flow circulation stimulates fluid mixing, hence exhibits inherent ability to promote wall heat transfer, even under laminar flow conditions. Clear understanding of the secondary flow mechanism is thus significant for the development of effective analytical tools for thermal and hydrodynamic design of technological systems having embedded curved geometries, such as gas turbines blades, air conditioning, steam boilers, coil heat exchangers and rocket engine coolant systems. This paper reviews the established knowledge-base in fluid flow and thermal behaviour in heated curved ducts, covering both experimental work and numerical simulations while appraising the adopted methodologies for analytical limitations. Extending the current understanding, the paper then provides the details of a carefully-planned research study for single and two phase fluid flows in curved ducts. In this, a novel numerical simulation is developed for single phase fluid flow in heated curved ducts using three-dimensional vortex structures (helicity) and a curvilinear mesh system that overcomes previous modelling limitations. Considering both rectangular and elliptical ducts, an extensive parametric investigation is performed by evaluating helicity, temperature and pressure profiles, while examining the influence from flow rate, wall heat flux and duct aspect ratio on the flow behaviour. Unique fluid and thermal characteristics in curved ducts are interpreted with physical reasoning while validating against limited experimental data. Two novel approaches are formulated for accurate detection and prediction of hydrodynamic instability in curved passages. These techniques are compared and assessed for their merits over previously published methods. An entropy-based analysis is developed to optimise thermal characteristics in curved ducts minimising pressure drop penalties while maintain high wall heat dissipation rates. The paper then focuses on a study of two-phase flow behaviour in curved ducts. For this more complex flow situation, the details are provided on the development of a novel three-dimensional simulation using Eulerian multi-phase approach, non-equilibrium vapour-liquid phase condition and multi-phase k-ɛ turbulent solver. This methodology significantly improves integrity of predicted results with considerable computational advantage. The model is tested for numerical stability and validated against reported experimental results. The flow regimes and temperature fields are obtained for flow boiling in curved pipes and compared with those in straight ducts with an analysis on observed differences in characteristics. The study evaluates individual wall heat flux components that make up the overall heat transfer, namely that from liquid evaporation, quenching, single phase liquid and single phase vapour. These are effectively used for illustration and physical interpretation of the unique effects from pipe curvature on the flow boiling process. The study also discusses the parametric influence from liquid sub-cooling, wall heat flux and centrifugal forces on flow patterns and, the onset of flow boiling in curved pipes along with the phase change and associated heat transfer.
Professor Tilak Chandratilleke is the Head of Mechanical Engineering Department at Curtin University, Perth, Western Australia. He obtained his PhD in two-phase flow and boiling heat transfer from the Cambridge University in the United Kingdom. Contributing to nuclear industry and space travel applications, his PhD research developed the fundamentals for vapour bubble growth and departure dynamics under zero and partial gravity conditions. He worked as a consulting engineer in UK and USA for several years prior to joining the academia, where he developed academic programmes of international standing. Through several innovative discoveries and patents in heat and fluid flows, he has significantly contributed to the academic knowledge, commercial product development and community at large. These include the thermoelectric refrigerator-freezer technology, high-performance micro-scale heat sink technology, novel pulsed-jet electronic cooling mechanism and heat shielding concept for regulating water utility pipe temperatures. His research focus extends to single and two-phase heat transfer in curved flow passages, microchannel heat transfer, electronic cooling technologies and thermal radiation. In these, he has developed highly-cited novel experimentation and numerical methodologies and established new research directions for these fields with extensive research publications. He serves on the editorial board of the Institution of Mechanical Engineers (UK) Journal of Power and Energy and is currently the national chair of the Australasian Thermal Engineering Society organising international heat and mass transfer conferences. As an executive member of the Engineers Australia Mechanical Panel (Western Australia), he contributes to the professional engineering development and continuing education. Prof Chandratilleke has been widely honoured for research innovation, academic leadership and industry product development.
Numerical Modeling of Asphaltene Deposition
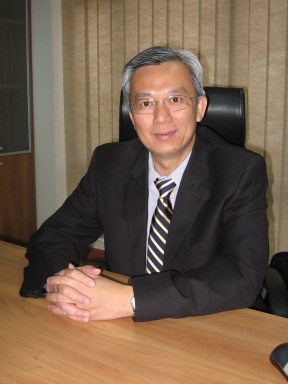
Prof John C Chai
The Petroleum Institute, UAE
Abstract: Deposition is a widely encountered engineering process. For examples, physical or chemical vapour deposition is employed to produce thin films for semi-conductor devices and optical coatings. Various surface finishing techniques are essentially electro-deposition. Deposition can have very undesirable effect. For example in the oil and gas industries, the deposition of wax, hydrates or asphaltene on the wall of the pipeline reduces flow of gas/oil and clogs the pipeline eventually if left untreated. For depositions to be employed or to be prevented, a thorough understanding and the ability to predict these deposition processes are therefore highly desirable. In this work, we present the modeling of a general particle deposition process on a fixed mesh. The two essential components in the model are (1) modeling of the mechanism involved in deposition process at the depositing front and (2) representation of the evolving depositing front. For the former, we consider the deposition of particles onto surfaces as a first order reaction. Extension to accommodate higher order reaction will be further elaborated. Two different front-capturing methods, i.e. the level-set method and the total concentration approach, are presented for the purpose of capturing the evolving depositing front. Fluid flow is modeled using the incompressible Navier-Stokes equations. Numerical solutions are demonstrated within the framework of a Cartesian finite volume method. Validations were conducted against a few solely diffusion driven one-dimensional and two-dimensional deposition problems with known exact solution. Finally, applications of these procedures to two-dimensional deposition problems with and without fluid flow coupled are presented.
John C. Chai is currently professor and chairman of the Department of Mechanical Engineering at the Petroleum Institute where his primary research interests are in the development of numerical methods for various transport phenomena. His main focus in recent years has been on numerical methods for multi-phase fluid flow and heat transfer. These include, but are not limited to, digital microfluidics, wet chemical etching and asphaltenes depositions. He received his B.S. (with First-Class Honors) in Mechanical Engineering from the University of Windsor, Canada and his M.S. in Mechanical Engineering from the University of Wisconsin-Milwaukee. In 1994 he graduated from the University of Minnesota with a Ph.D. in Mechanical Engineering where he worked under the supervision of Prof. Suhas V. Patankar. He has published 70 journal articles and contributed a chapter to the second edition of the Handbook of Numerical Heat Transfer. He is an Editorial Board member of Computational Thermal Sciences and an ASME Fellow.
Designed Optimum Geometry for Micro-channel and MIcro Pin Fins Heat Sinks Cooling Technology

Prof Tunde Bello-Ochende
Department of Mechanical and Aeronautical Engineering, University of Pretoria
Abstract: This paper reports on the theoretical and geometric optimization of micro-channel heat sink and micro pin fins. Different types of micro-channel heat sinks and pin fins configuration were studied. In all the cases the objectives is to maximize the global thermal conductance subject to a fixed volume, high conducting material and a fixed pressure drop. In the first configuration the micro-channel is completely embedded inside a high conducting material and numerical simulations were carried out on a unit cell with volume ranging from 0.1 to 0.9 mm3 and pressure drop between 10 and 75 kPa. The axial length of the micro-channel heat sink was fixed at 10 mm. The cross-sectional area of the micro-channel heat sink is free to morph with respect to the degree of freedoms provided by the aspect ratio and the solid volume fraction. The effects of the total solid volume fraction and the pressure drop on the aspect ratio, channel hydraulic diameter and peak temperature are investigated. In the second configuration the micro-channels are embedded inside a high conducting material, except that the top is covered with an insulating material. The whole configuration was allowed to morph with respect to all the degrees of freedom. Similar but dimensionless numerical simulations were carried out on this configuration and the numerical optimization results were reported. In the first configuration numerical results show that the degrees of freedom have a strong effect on the peak temperature and the maximum thermal conductance. The optimal geometric characteristics (aspect ratio and the optimal channel shape) are reported and compared with those obtained from approximate relationships using scale analysis. For this configuration the predicted trends are found to be in good agreement with the predicted results. In the second configuration a test case on an actual micro-channel heat sink shows a reduction of about 8% in global thermal resistance. In the third part of the report use constructal theory to discover the geometry and spacing between two rows of pin fins rows so that the total heat transfer rate is maximized. The heat transfer across the fins is by laminar forced convection bathed by a free-stream that is uniform and isothermal. The optimization is subjected to fixed total volume of fin materials. The dimensions of the optimized configuration are the result of balancing conduction along the fins with convection transversal to the fin. The resulting flow structure has multiple scales that are distributed non-uniformly through the flow structure. The results predicted based on scale analysis are in good agreement with the numerical results. The results also show that the flow structure performs best when the-fin diameters and heights are non-uniform.
Prof Tunde Bello-Ochende is currently an Associate Professor at the Department of Mechanical and Aeronautical Engineering, University of Pretoria. His research focuses on the theoretical, experimental and numerical optimization of heat transfer devices from macro to micro scales. These include compact heat exchanger, micro-channel heat sink, and heat transfer augmentation using micro-fins. His recent work are in the areas of sustainable/renewable energy (solar, geothermal and fuel cells). He is the author of over 55 papers in referred journals and proceedings. He is a member of America Society of Mechanical Engineer (MASME). Prof. Bello-Ochende received his Bachelor’s, Master’s degree from the University of Ilorin (1995, 1999) and doctorate from Duke University (December 2004), all in Mechanical Engineering.
Designed Optimum Geometry for Micro-channel and MIcro Pin Fins Heat Sinks Cooling Technology
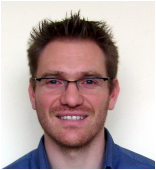
Professor R Revellin
National Institute of Applied Sciences of Lyon
Abstract: This paper aims at presenting the latest scientific progress on two-phase flow in singularities through academic research at INSA Lyon as well as proposing some future possible important issues to be investigated. Flow regimes of third- and fourth-generation refrigerants in horizontal and vertical return bends as well as in a horizontal sudden contraction were experimentally investigated. The dynamical behavior of vapor bubbles or slugs in vertical downward flow return bend was reported. A simplified analysis of the forces acting on the bubble was proposed to better understand the vapour trajectory. Furthermore, void fraction was measured along the sudden contraction using an image analysis technique, which gives very original results. Such experimental studies also brought to the fore the upstream and downstream flow disturbances caused by such singularities as contractions and return bends and their impact on the hydrodynamic performance (e.g. pressure drop) of refrigerants. Especially, these disturbances can be analysed in terms of perturbation lengths up- and downstream of the singularities. Lastly, large pressure drop databases for R-410A, R-134a and HFO-1234yf were obtained. Experimental values of pressure drops in singularities were compared against different prediction methods from the literature without any satisfactory results. Finally, these databases were used to develop new two-phase pressure drop prediction methods for such singularities as return bends and sudden contractions.
Rémi Revellin is Associate Professor at the National Institute of Applied Sciences of Lyon (INSA Lyon) in France since 2007 and is currently working at the Center for Thermal Sciences of Lyon (CETHIL). He is also the head of the international academic affairs for the Department of Energy and Environmental Engineering at INSA Lyon. He teaches thermal systems engineering thermodynamics, convective heat transfer and phase-change heat transfer at the Department of Energy and Environmental Engineering at INSA Lyon.
His primary interests of research are: two-phase flow and flow boiling in macro and microchannels, micro-heat pipes, constructal theory and thermodynamics (at equilibrium and at non equilibrium state). He has published more than 30 journal papers on these topics as well as a few chapters in edited books.
He obtained his PhD in 2006 at the Swiss Federal Institute of Technology in Lausanne (EPFL). He received the Eurotherm Prize 2008 for his work on the experimental two-phase fluid flow in micro channels.